Fundamentals
of the Stabilization of Soils With Lime
Dallas N. Little
Professor of Civil Engineering
Texas A&M University
Summary
Lime is an effective
stabilizer for a wide range of soils. Actually, two phases of stabilization
occur in a lime-soil system. The first involves the practically immediate
reactions of cation exchange and flocculation-agglomeration. These
reactions accur to some extent with all fine-grained soils. Due to
textural changes caused by these reactions within the soil., the strength
and moisture stability of these soils is improved. These improvements
are reflected in improved workability, imediate strength improvement
and reduced swell-susceptibility.
Pozzolanically
induced long-term strength gain is more capricious as its succes depends
upon a cooperative reaction between the lime and the clay as well
as the mineralogy of the clay. Many clays are reactive and upon lime
stabilization their strength may easily triple or quadruple. In some
instances strengths have improved by an order of 10 or more.
Lime, the versatile
soil stabilizer, should be considered with all soils when the plasticity
index exceeds 10 percent and the percent of soil smaller than the
number 200 sieve exceeds 25 percent.
Need
for Stabilization
Soils often require
stabilization to add mechanical stability, to improve durability or
to alter their volume change potential. The mostwidely recognized
form of stabilization is compaction, which improves the machanical
stability of virtually any soil. However, compaction alone is often
not enough. Thsi is especially true with fine-grained, cohesive soils.
Plastic clays
pose a unique problem to the enginer. Their consistency varies over
a very wide range. This consistency is directly related to the availability
of water. The ability of clays to take-on and lose water is a function
of their morphology and mineralogical nature.
Lime (either quicklime
or hydrated lime, in both high calcium anddolomitic types) is an effective
stabilizer of clays. The lime actuallyalters the ability of the clay
to hold water at its surface and can react with the clay to procedure
a cement which may add substantially to the strength of the lime-stabilized
clay.
This paper discusses
the unique phenomena which occur when lime and clay are mixed. These
phenomena result because of the unique mineralogy of clays and the
chemical properties of the calcium and/or magnesium compounds present
int he lime.
Nature
of Soil
One normally differentiates
soil into groups according to particlesize. In fact, the Unified Soil
Classification System divides soils into categories of gravels, sands,
silts and clays, based on size. A look at the mineralogy of soils,
however, shows that differentiation according to size is only a beginning
in understanding soil behavior.
Soil
Mineralogy
A course in soil
mineralogy provides an excellent background for an engineer seeking
to understand the phenomena involved in soil stabilization. The subject
of soil mineralogy is complex and intricate. For our purposes, a simple
comparison of the mineralogical structure of a few commonly ancountered
soils will illustrate the critical role of mineralogy and crystal
structure.
The Earth’s
crust to a depth of about 10 miles is composed mostly of oxygen (47,3%),
silicon (27,7%). These are followed by smaller amounts of the metals
iron (4,5%), calcium (3,5%), sodium (2,5%), potassium (2,5%) and magnesium
(2,2%). The manner in which these elements combine to from the compounds
which form the soils and rock sin the crust is affected by the thermodynamics
of the genesis process. Organized atomic arrangements don’t
just happen but occur in a fashionwhich will preserve electrical neutrality,
satisfy bonding directionality, minimize strong ion repulsions and,
in short, provide the most stable arrangement of atoms possible.
Two of the most
common building blocks are shown in Figure 1. These are the silica
tetrahedron and the aluminum octahodrom. Within each building block
the multivalent cation is coordinated with oxygen. Thus the elements
comprising approximately 83% of the arth’s crust are accounted
for by these two basic building blocks.
The silica tetrahedron
represents the basic coordination polyhedron which forms the prolific
minerals quartz and feldspar. The silica tetrahedron is not electrically
neutral. Tetrahedra link together in arrangements which minimize strong
repulsions between the silicon ion. The high positive charge of the
silicon ion develops a variety of possible packing arrangements in
response to the repulsions generated between adjacent cations. Figure
2 shows one such arrangement of these tetrahedra-the tree-dimensional
space lattice silicate.
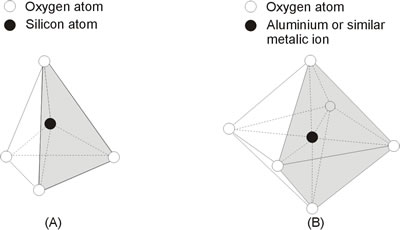
Figure 1. Two
of the most common building blocks of soils: (a) silica tetrahedron
and (b) aluminum octahedron.
The space-lattice
silicate, or framework silicate, result when all four oxygen atoms
are shared with other tetrahedra areactually grouped to form a spiral.
The sharing of oxygen atoms among the tetrahedra results int he strongest
type of chemical bonds among the tetrahedra-primary valence bonding.
A framework silicate
like quartz is a very strong (mechanically) and durable (mechanically
and chemically) mineral. This stability i sdue to the very stron internal
bonding.
Another framework
silicate almost identical to quartz is feldspar. Int he formation
of feldspar, part of the silicon positions are filled by aluminum.
Because aluminum has a lower charge by +1 than silicon, a charge imbalance
within the three-dimensional structure result. This charge potential
is balanced by cations adsorbed within the lattice structure such
as potassium, calcium and sodium. The feldspar mineral is weaker and
less durable than quartz for two reason: (1) the aluminum cation is
larger than the silicon ion and does not fit among the oxygen atom
sas precisely as does the silicon and (2) the adsorbed cations within
the three-dimensional silicate make the structure more susceptible
to weathering.
Despite the dissimilarities,
both quartz and feldspar result in particles which are equi-dimensional,
granular, hard and chemically relatively stable. Quartz and feldspar
minerals may exist over a wide renge of particles sizes from gravel-size
to silt-size. In fact quartz, due to its extremely stable nature,
may even retain its mineralogical structure when weathered down to
clay-sized particles (less than 2 microns in size). Ont he other hand,
feldspar, because it is less mechanically and chemically stable, does
not retain its basic mineralogical structure upon extreme weathering.
In otherwords, one would not generally find feldspar minerals of a
clay-size.
The three-dimensional
framework silicates are examples of non-plastic, granular soils.
The
Clay Minerals
Silica tetrahedra
are joined together only at their corners because of strong repulsion
between adjacent triangles of the tetrahedra. This arrangement allows
the tetrahedra to form several crystal arrangements in addition to
the framework silicates. Included in these structures are independent
silicates, ring sor chains of tetrahedra, and sheet silicates. The
sheet silicates are one unit thick but theoretically may expand infinitely
int he lateral dimension. These are the units from which the clay
minerals are formed.
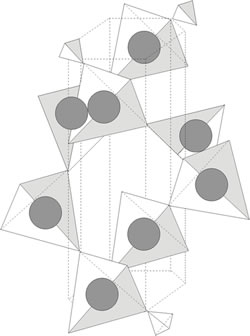
Figure 2. Three-dimensional
arrangement of silica tetrahedra typical of quartz – a stable,
equi-dimensional mineral. Note the peak-to-peak coordination of tetrahedra
where the bonding is vera powerfull primary valence bonding (After
Moffatt et. Al., 1965).
A typical scenario
for the formation of clay minerals is the chemicalweathering of feldspar.
Hydrolysis is probably the most importantchemical weathering process
and is caused by a reaction between the ions within the feldspar mineral
and the dissociated hydrogen (h+) and hydroxyl (OH-) ions of the water.
The small h+ ions
dissociated from the water molecule can easily enter the open lattice
structure of the feldspar mineral and, in great concentrations, they
will replace metallic ions within the lattice which have been adsorbed
to neutralize the change deficiency caused by the substitution of
aluminum for silicon during the formation of the feldspar. The replaced
ions include sodium, potassium and calcium.
Next, the hydrogenated
surfaces of the mineral become unstable, and sheets of tetrahedra
and octahedra are formed and peel off. These tetrahedral and octahedral
sheets are illustrated in Figure 3.
The two basic
sheets, tetrahedral and and octahedral, shown in Figure 3 are stacked
together to form the most prominant clay minerals. Two common arrangements
of tetrahedral and octahedral sheets are presented in Figure 4. The
1:1 configuration is typical of the mineralKaolinite which has low
plasticity. The 2:1 configuration is typical of the clay mineral smectite
which can be very plastic and unstable.
In both configurations,
a plane of atoms is common to both the tetrahedral and octahedral
sheets. The bonding among the sheets is very strong primary valence
bonding. However, the bonds that link the unit structures together
to form particles are much weaker and are the reason for the variable
response of the minerals in terms plasticity and consistency. Before
the types and characterics of linkages between units can be discussed,
the phenomena of surface charge of the unit cells must be explained.
During the genesis
of some clay minerals a phenomena called iso-morphous substitution
occurs. In this process some of the silicon ions in the tetrahedral
sheet or aluminum ion sin the octahedral sheet are proced by other
metallic ions of a lower positive charge (lower valence). The result
is a charge deficiency which is reflected in a net negative charge
at the surface of the clay unit cell. Some minerals, such Kaolinite,
experience very little isomorphous substitution. In Kaolinite, about
one in every 400 silicon atoms (+4 valance)., are replaced by aluminum
(+3 valence) int he tetrahedral sheet. Smectite, ont he other hand,
experiences abundant isomorphous substitution, about one in six aluminum
atoms (+3 valance) int he octahedral sheet are replaced by magnesium
(+2 valence). The result is that smectite minerals have a very hogh
negative surface charge and Kaolinite minerals have a low surface
charge. The ratio of surface charge between smectite and Kaolimite
is about 10 to 1.
7
oldal grafikak
Figure 3. Basic
sheet arrangements of (a) silica tetrahedra and (b) aluminum octahedra.
Each sheet is one unit thick but may theoretically expand without
bound int he lateral direction.
8
oldal grafika
Figure 4. Basic
units of the 1:1 mineral Kaolinite (a) are linked with relatively
strong hydrogen bonds which retain a high degree of moisture stability
among layers while the basic units of the 2:1 smectite mineral (b)
are linkedby weak cation attraction. The efficiency of this linkage
is a function of the type and concentration of the available cations.
The manner in
which the basic unit cells of the clay minerals are linked together
is strongly affected by the surface charge as well as the mineral
structure. Kaolinite unit cells are linked by hydrogen bonds between
oxygen atom sin the base of the tetrahedral sheet and hydroxide ions
at the surface of the octahedral shhet, Figure 4. Hydrogen bonding
is a secondary valence bonding and is not nearly as strong as the
primary valence bonds that link the sheets together. However, the
bonding is strong enough to prevent the infiltration within the layers
of water or foreign particles, such as cations. The result is that
Kaolinite is a relatively stable clay of low plasticity.
The large amount
of isomorphous substitution within smectite unitsyields a clay mineral
with a substantially negative surface charge. This charge is satisfied
by the adsorption of positively charged ions, cations, at the surface.
Linkage between successive layers is due to the cations which balance
the surface charges. This cation linkage is a very weak bonding and
results in particles of clay with prominent planes of cleavage or
weakness. Due to the weak inter-unit bonding, smectite clayparticles
are much smaller particles than kaolinite particles.
The response of
the smectite clay mineral to water is highly dependent upon the ions
available int he pore water. Thus the ability to swell and shrink
and demostrate plasticity is controlled by the clay-water interaction.
Clay-Water
system
To this point
we have established that clay particles are generally conposed of
tetrahedral and octahedral sheets. Int he smectite mineral, the 2:1
arrangement, Figure 4, coupled with a substantial level of isomorphous
substition, result in clay particles which have a platelike morphology.
These particles are also very small and possess enormous surface area.
In fact, smectite minerals often have surface areas approaching 800
m2/gm. This may be compared to only about 15 m2/gm for Kaolinite.
With this enormous available surface area coupled with the highly
charged nature of the clay surface, it is no wonder that the clay
is so active and can so readily adsorb polar liquids like water and
cations available int he enviroment.
Many explanations
of the clay-water system are available. Some arequite complex. Figure
5 is an attemp to simplify the explanation while accounting for the
important phenonema. First, in Figure 5a the negative clay surface
is shown to be surrounded by positively charged cations attracted
to the surface to equilibrate the charge potential. These ions are
not orderly arranged at the clay surface but form a diffused layer.
This diffusion is due to like charge repulsion and thermal agitation
of the cations. A second phenomenon illustrated in Figure 5a is the
diffusion of water molecules toward the high concentration of cations.
The inward diffusion is triggered by the desire of nature to move
to a more random (less ordered) condition.
Grafika
10 oldalrol
Figure 5. Cations
and water (a dipolar melecule) are attracted to the negatively charged
clay surface to satisfy the charge potential. This results in (a)
adsorbed cations and water molecules and (b) a diffused layer of cations
due to their thermal activity and the infusion of water toward the
clay surface because of the high electrolyte concentration (After
Mitchell, 1976).
The water molecules
not only seek diffuse the absorbed cation layer buta re actually attracted
to the cations and to the negative clay surface due to their unique
dipolar structure. In Figure 5b, the water molecules are represented
as molecules with distinct positive and negative sides due to the
molecular arrangement of the hydrogen and oxygen atoms. Some researchers
believe that a layer of water molecules is attached to the clay surface
by hydrogen bonding and that subsequent layers are more loosely held.
This is illustrated in Figure 5b. At anyrate, the net result is a
diffused layer surrounding the clay comprised of (1) water held by
hydrogen bonding and/or attracted by the diffusion gradient and (2)
a diffused layer of cations, which are attracted to the negative clay
surface, and anions (negatively charged ions) which are attracted
to the cation and dipolar water molecules.
The net result
of a highly charged surface, as is the case with smectitic clays,
coupled with „unfriendly” cations, which have a single
plus charge per ion and are thermally active, is a highly diffused
water layer surrounding the clay particles. Because of this layer
some smectites have been documented to hold seven times their dry
weight in absorted water. When fully hydrated these diffused water
layers force the clay platelets into a parallel arrangement which
offres very little shear strength.
The thickness
of the diffused water layer is greatly dependent upon the type and
concentration of cations available int he pore water. Divalent cations
(cations with a 2+ charge) can much more efficiently solve the negative
charge potential than can monovalent cation (cations with a 1+ charge).
Thus, the resulting diffused divalent cation-water layer around clay
particles is much smaller than the duffused monovalent cation-water
layer around clay particles of idential mineralogy.
The
Dramatic Change: The Lime-Clay System
A Stable Water Layer
Lime for use in
soil stabilization is most commonly produced as either hydrated high
calcium lime, monohydrated dolomitic lime, calciticquicklime or dolomitic
quicklime. When lime is added to a clay-water system, the divalent
calcium cations yirtually always replace the cations normally adsorved
at the clay surface. This cation exchange occurs because divalent
calcium cations can normally replace cations of single valence, and
ion sin a high concentration will replace those in a lower concentration.
The fact that
calcium will replace most cations available in the water system is
documented by the Lyotropic series which generally states that higher
valence cations replace those os a lower valence, and larger cations
replace smaller cations of the same valence. The Lyotropic series
is written as: Li+ < Na+ < H+ <K+ < NH4+ << Mg++
< Ca++ << Al+++ where the cation to the right replaces the
one to the left. Tgus inequal concentrations, Ca++ can easily replace
the cations commonly present in most clays.
Gapon (Yong and
Warketin, 1966) explains that the relationship between the cations
adsorbed at the clay surface is a function of not only the concentration
of cations but also the valence. Gapon’s most simple and useful
equation states:
12
oldal egyenlet
where M and N
are cations of valence m and n, respectively, and e refers to exchangeable
and o to ions in the outside solution. The constant kdepends on the
specific cation adsorption effects and upon the clay surface. Based
on this equation, equal concentration of Ca++ and Ca++ ions in the
outside water, natural pore water, will result in 17,5 times more
Ca++ ions present at the clay surface than Na++ ions. The dual effects
of divalency of the calcium ion and very high concentration which
would result from the addition of lime to a soil-water system are
obvious.
A
New Texture
The effect of
exchangeable cations on the size of the diffused water layer is illustrated
in Figure 6a and 6b. Cation exchange due to the addition of lime result
in stabilization of the diffused water layer and a dramatic reduction
in its size. When the clay particles are allowed to approach each
other more closely due to reduction in the size of the water layer
an edge-to-face attraction or floccuation occurs. Flocculation is
additionally enhanced due to a electrolyte concentration and high
pH enviroment existing in the lime-soil-water system. The edge-to-face
attraction is probably partly due to the attraction of broken bonds
at the edge of the clay particles to the oppositely charged surfaces
of neighboring clay particles.
The net result
of cation exchange and flocculation/agglomeration of particles is:
1. Substantial
reduction and stabilization of the adsorbed water layer
2. Increased internal friction among agglomerates and greater aggregate
shear strength.
3. Much greater workability due to the textural change from a plastic
clay to a friable, sand-like material.
13
oldal a grafika
13oldal b grafika
Figure 6. The
reason for the textural change is due to the phenomenon of cation
exchange followed by flocculation and agglomeretion. Figure 6 (a)
illustrates low strength clay soil where particles are separeted by
large water layers. The addition of lime (calcium) shrinks the water
layer (b) allowing the plate-like particles to flocculate.
Immediate
Strength Improvement
Laboratory evidence
substantiates textural and property changes due to cation exchange
followed by flocculation/agglomeration. Table 1 illustrates the ability
of relatively small percentages of lime to reduce the plasticity index
and swell potential of plastic, troublesome clays to innocuous levels.
Figure 7 illustrates the increase in shear strength, as measured by
the California Bearing Ratio (CBR) of a low plasticity clay due to
textural changes in the clay. These textural changes are due to cation
exchange followed by flocculation/agglomeration, and these changes
essentially as occur as rapidly as the lime can be intimately mixed
with the clay. The soaked CBR tests (96 hour soak) in Figure 7 were
performed immediately after compaction, without long-term cure effects.
Table 1. Attemberg
Limits for Natural and Lime-Treated Soils (After Thompson, 1967).
14
oldal táblázat
The reason for
the improved shear strength is illustrated in Figure 6 where an unaltered
clay whose diffused water layer is hydrated is compared to the same
clay after lime stabilization. The structure of the clay platelets
surrounded by the hydrated, diffused water layers provedes very little
shear strength. The only resistance to relative movement is due the
overlapping and interferences among the water layers. On the other
hand, in the flocculated structure, the summation of the edge to face
contacts provides a more substantial sear strength.
Long
Term Strength
The phenomenon
of cation exchange and the concomitant textural changes occurs with
all clays. Of course, the degree of effect and/or amount of lime rquires
to cause cation exchange is based on the chemical and mineralogical
conditions of the soil and the water environment.
15
oldal grafikon
Figure 7. CBR-Moisture content relations for natural and lime-treated
(3% 5%) CL Soil (AASHTO T compaction) (After Thompson, 1970).
The long-term
strength is more complex and is heavily influenced by soil conditions
and mineralogical properties. However, many clay soils arepozzolanically
reactive when stabilized with lime and respond with anappreciable
strength gain due to the development of a cemented matrix among the
soil particles.
A pozzolan is defined as a finely divided silicions or aluminous material
which in the presence of water and calcium will form a cemented product.
The cemented products are calcium-silicate-hydrates and calcium-aluminate-hydrates.
These are same hydrates that form duringthe hydration of Portland
cement.
Clay is a pozzolan
as it is a source of silica and alumina for the pozzolanic reaction.
Clay-silica and clay-alumina become soluble or available in a high
pH enviroment, Figure 8. The pH of water saturated with lime is 12.45.
Thus a soil-lime-water system has a pH hogh enough to solubilize silica
and alumina for pozzolanic reaction. As long as enough residual calcium
remains in the system to combine with the clay-silica and clay-alumina
and as long as the pH remains high enough to maintain solubility,
the pozzalanic reaction will continue. The reaction is illustrated
by the following equations:
Ca++ OH- + Soluble
Clay Silica – Calcium Silicate Hydrate (CSH)
Ca++ OH- + Soluble Clay Alumina – Calcium Aluminate Hydrate
(CAH)
Eades and Grim
(1966) skillfully adopted the pH increase phenomenon in a design procedure
for lime-soil mixtures. Their procedure requires for succifient lime
to be added to the soil to satisfy all immediately occuring reactions,
and yet provide enough residual lime to maintain a pH of 12,4 for
sustaining the strength-producing reaction.
What is unique
about the pozzolanic phenomenon is the cooperative reaction between
the lime and the clay. The lime induces the high pH environment which
solubilizes the silica and alumina. The lime also provides the residual
free calcium which combines with the silica and alumina supplied by
the clay to produce the pozzolanic reaction.
Evidence of the
strength of the pozzolanic reaction comes from both field and laboratory
data. Strength increases of greater than 100 psi can be achieved with
many soils following 28 day curing at temperatures of approximately
70oF. Extended curing either in the laboratory or under field conditions
may produce strength increases of several hundred psi.
Field data indicate that with some soil-lime mixtures strength continues
to increase with time up to in excess of ten years.
The Mohr-Coulomb
criteria is often used to evaluate the shear strength of granular
materials. This criteria states that shear strength is provide by
(1) the cohesive strength of the soil and (2) the strength due to
internal friction. Mathematically the law reads:
Shear Strength
= Cohesive Strength © + Normal Stress (N) x tan Ø
Where Ø is the angle of internal friction.
17
oldal grafika
Figure 8. Effect
of pH on the solubility of clay silica and clay alumina (After Keller,
1964).
Typical angles
of shearing resistance or internal friction for lime stabilized clays
are between 25o F and 35o F. This may be as much as 100% higher than
for the natural clay soil . This component then adds to the shear
strength more as the confining pressure ont he soil increases. The
cohesion valum (c) increases with the conpressive strength of the
mixture. A rough estimate of c is 30 percent of the unconfined compressive
strength (Little, et. Al.,1987).
Stiffness and
load spreading capability of the stabilized clay also increase with
development of pozzolanic strength. Field deflection data recorded
by Texas A&M University has shown that resilient moduli of plastic
Texas clays in the Houston area have been increased from between 5,000
and 10,000 psi for the natural clay to between 20,000 psi and 70,000
psi after lime stabilization. Other researchers have documented stiffnesses
of over 100,000 psi for lime stabilized clays (TRB Crcural, 1976).
Suitable
Soils for Lime Stabilization
Experience has
shown that lime will react with medium, moderately fine and fine-grained
soils to produce decreased plasticity, incrreased workability, reducer
swell and increased workability,reduced swell and increased strength.
Generally speaking, those soils classified by the Unified System as
CH, CL, MH, SC, SM, GC, SW-SC, SP-SC ,SM-SC, GP-GC, GM-GC arepotentially
capable asbeing stabilized with lime.
The key to a pozzolanic
reaction resulting in long-term strength gain is the presence of a
reactive clay to provide the pozzolans. Although lime cannot react
pozzolanically whit sands (composed of framework silicates9 whitch
have no clay fraction, lime may be an effective stabilizer with sandy
or silty soils which have a clay content as low as sever percent and
a plasticity index as low as 11(Little,et,al.,1987).
As a general guide
to stabilization (Little,et.al.,19879 proposed that lime stabilization
should be considered as the primary stabilizer or at least as a pre-stabilizer
for all soils having plasticity indices of greater that 10 and greater
that 25 percent of the shoil smaller that the number 200 sieve. In
the case of plasticity indices above 30 and greater that 25 percent
material passing the number 200 sieve, the selection criteria recommended
by Litle, et. al. (1987) for use by the Air Force requires the use
of lime or lime as a pre-treatment to reduce the plas ticity index
below 30 followed by Portland cament stabilization.
The extent which
the soil-lime pozzolanic reaction proceeds is influenced primarily
by natural soil properties. With some soils, the pozzolanic reaction
is inhibited, and cementing agents are not extensively formed. Those
soils that react with lime to produce substantial strength increase
(greater than 50 psi following 28 day curing at 73o F )are ”are
”reactive” and those that disply limited pozzolanic reactivity
(less than 50 psi strength increase) are ”nonreactive”
(Thompsom, 1970).
The majorsoil
properties and characteristics which influence the lime-reactivity
of a soils, i.e.,ability of the soil to react with lime to produce
cementitious materials, are soils pH, organic carbon content, natural
drainage, presence of excessive quantitites of exchangeable sodium
,clay mineralogy ,degree of weathering ,presence of carbonates,extractableiron,
silica-sesquioxide ration and silica-aluminal ratio.Itis emphasized
that the main factors controlling the development of pozzolanic cementing
in a lime treated soil are the inherent properties and characteristics
of the soil. If a soil is ”nonreactive”, extensive pozzolanic
strengthdevelopement wil not be achieved regardless of lime type,
lime percentage or curing conditions of time and temperature.
Soil-lime reactions
are complexand not completely understood at this time. However, sufficient
basic understanding and successful field experience are available
to provide the basis of an adequate technologyfor successfully utilizing
soil-lime stabilization under a wide variety of conditions.
Thompson (1970)has
published data, Table 2, which demonstrates the wide-randing pozzolanic
reactivity which can occur with fine-grained soils.
Table 2. Compressive
Strength Data for Natural and Lime-Treated Soils (After Thompson,
1970).
19
oldal tablazat