Improvement
of clay soils in situ using lime piles int he UK
C.D.F. Rogers, S. Glendinning
Abstract
Lime piles, which
essentially consist of holesin the grounfd filled with lime, have
been used for two distinct purposes for the treatment of clay soils
in situ. The first concerns the treatment of soft soils to improve
their bearing capacity and in this case uses relatively large diameter
quicklime piles at close spacings. The result is a significant reduction
int he water content of the soil, causing densification and concomitant
increases in its strength and stiffness. The secondapplication ia
int he stabilisation of failing slopes, for which both quicklime and
lime slurry piles have been used withthe intention of causing ion
migration and subsequent lime-clay reactions int he surrounding soil.
However, although
they have been successfully used worldwide, their usage has been relatively
limited in relation to other techniques and the applications in which
they have been used diverse. For this reason the literature ont he
subject has tended to be inconsistent at best, and in some cases directly
contradictory. There is thus an apparent lack of understanding of
how lime piles work. This paper aims to produce some clarity by interpreting
the literature int he light of recent research.
A summary of the
stabilisation mechanisms that the current authors believe to operate
is presented and the evidence from the literature that supports or
contradicts these mechanisms is discussed. The result of this process
thus provide a basis of design, albeit using parameters that need
further definition for site specific application. For illustration,
the design process in discussed in terms of UK application. ©
1997Elsevier Science B.V.
Keywords: Lime
stabilisation; lime piles; Lime columns; Soft soil treatment; Ground
improvement; Spole stabilisation
1.
Introduction
Clay presents
problems to geotechnical engineers due to its complex nature. This
derives from its plasticity, its low permeability and thus the time
dependency of pore water pressure ( hence shear strength) change and
volume change, its structure, and its chemistry and mineralogy. Two
particular facets of its behaviour that cause problems are its propensity
to shear and/or compress excessively when stressed, and its change
in stability in cuttings or embankment slopes with time. Improvement
of the clay by treatment in situ to a considerable depth is nessecary
if the often more expensive structural solutions to such problems
(piles, ground anchors, nails) are to be avoided. Chemical alternation
of clay has been shown to be effective in increasing its strength
and stiffness and, when combined with long-term cementing reactions,
considerable improvements can be achieved. Lime provides one such
means.
The use of lime
to improve the engineering properties of clay soils is well established,
if not fully understood. The basis of lime treatment is firstly cation
exchange (modification) followed by a reaction with the siliceous
components of clay to cause stabilisation (TRB, 1987). Modification
occurs within 24-72 h of mixing lime with clay and is manifested by
rapid flocculation of the clay particles. This fundamentally changes
its „cohesive nature” to a friable and granular nature,
and itsstrength is considerably improved ( Rogers andGlendinning,
1996a). Stabilisation concerns the long-term additional strength development
caused by crystallisation of calcium silicate hydrate and calcium
aluminate hydrate gels that form following the dissolution of clay
minerals in a highly alkaline environment.
Lime has been widely used in road construction by intimate mixing
with clay subgrades to improve workability, shear strength and bearing
capatity. Its use as a deep stabilising technique is less common,
although three discint methods exist. In China, Japanb, Singapore
and Scandinavia, for example, lime columns have been used to prepare
soft ground for foundations. The general principle of the technique
is to create, in situ, colums of intimately mixed lime and clay (Fig.
1.), which react to produce colums of material of greater strength
and (initially) permeability than the surrounding soil.
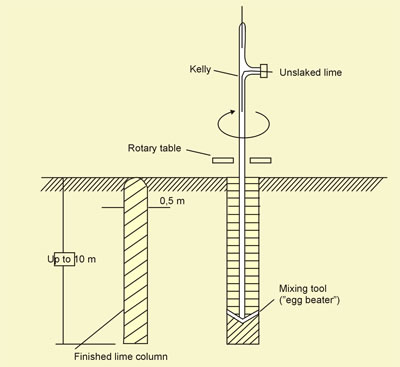
Fig. 1. Procedure for construction of lime columns (after Broms and
Boman, 1979).
Lime slurry pressure
injection, developed int he USA (Blacklock and Wright, 1986), uses
specialistequipment (Fig. 2). It has been used to treat expansive
clay soils, silts other soft ground, as well as failed embankment
slopes, although the problem of induced pore water pressures could
exacerbate stability problems.
The third technique concerns lime piles, which consist, very basically,
of holes int he ground filled with lime. Lime piles have been used
int the USA, Thailand, Sweden and Austria as a method of slope stabilisation
and in China, Japan and Russia as a ground improvement technique for
soft soil.
This paper addresses deep stabilisation of clay using lime piles.
The paper aims to summarise and discuss the experimental and field
data presented int he literature. These are interpreted in the light
of the stabilisation mechanisms postulated by ongoing research at
Loughboroungh University, the experimental programme for which is
reported elsewhere (Glendinning, 1995, and Rogers and Glendinning,
1994). Thereafter the potencial of the technique is illustrated in
terms of its potential application int he UK by way of general illustration.
2.
Construction and modus operandi of lime piles
Ingles and Metcalf
(1972) show one method of lime pile construction, illustrated in Fig.
3, in which a hollow tube is pushed into the soil to the required
depth and quicklime is forced into the tube under pressure it is withdrawn.
The pressure forces open the end of the tube allowing the lime to
fill the cavity below. After each metre isfilled, the end of the tube
is closed and used to compact the lime forming the pile. The altarnative
method pf construction in a self-supporting soil is to auger holes
to the required depth and subsequently, or via a central stem, to
add quicklime and compact in layers. In some cases hydrated lime,
or more commonly lime slurry, is added to the augered holes depending
ont he application and perceived stabilisation mechanism.
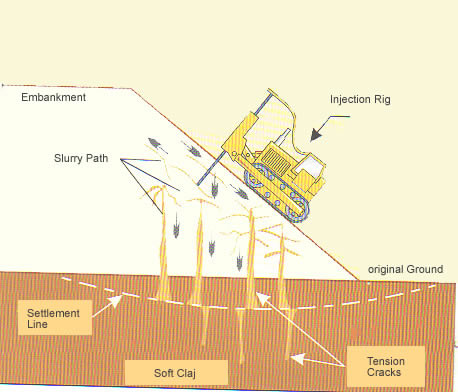
Fig. 2. Slope stabilisation using lime slurry pressure injection (after
National Lime Association, 1985).
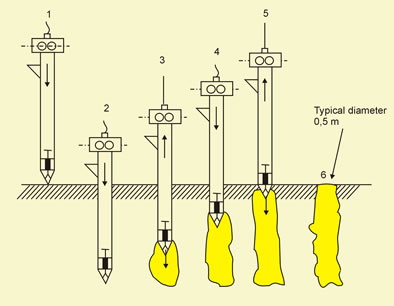
Fig. 3. Procedure for construction of lime piles in soft soils ( after
Ingles and Metcalf, 1972).
It should be noted
that the term „lime column” and „lime pile”
have been used interchangeably int he literature, resulting in confusion
over stabilisation mechanism. Indeed, different mechanismsoperate
with different combinations of construction technique and application.
Following experimental and field studies at LoughboroughUniversity,
the current authors believe the stabilisation mechanisms to be:
2.1
Lateral consolidation
As quicklime draws
in water from the surrounding ground it reacts, or slakes, to form
hydrated lime:
CaO+H2O ? Ca(OH)2
Considerable expansion
of the solid phase occurs if the quicklime is unrestrained since the
relative volume of quicklime to slaked lime is 1:1.99. Thisexpansion
is said to cause lateral consolidation of the ground surrounding the
pile. However consid erations of stoichiometry of the hydration reaction
as a whole (i.e. including the liquid phase) indicate that there is
a small net volume loss. Thus the idea of physical expansion cannot
be valid unless there is an external source of water causing hydration
of the lime. Densification of the assemblage of soil particles will
occur as a result of this process, since water is drawn out from between
them to react with the quicklime. Howewer, Densification as a result
of physical expansion causing water to be squeezed from the soil is
believed not to take place as a result of the above logic.
2.2
Water content reduction
The slaking reaction
„uses uo” some water from the surrounding soil and, being
highly exethermic, it creates steam. These combined processes are
thought by some authors to cause a significant water content reduction
Howewer, relatively large volumes of lime need to be added to achieve
significant water content reductions in cases where steam generation
is absent.
2.3
Clay-lime reaction
It is widely reported
that lime migrates from the piles and reacts with, and hence stabilises,
the surrounding (clayey) soil. For this to occer both calcium and
hydroxyl ions must migrate through the clay. Saturation of the system
by calcium ionsis nessecary for maximum cation exchange ont he available
exchange sites of the clay minerals. Hydroxil ions produce the highly
alkalinic (pH>12.4) conditions that are required for dissolution
of silica and alumina prior to the formation of calciom silicate hydrate
and calciumaluminate hydrete, the gels that crystallise to causestabilisatino.
In incatc clay the migration distanceshave been found to be relativelly
small (Rogers and Glendinning, 1996b).
2.4
Reduction in pore water pressure
The addition of
quicklime to a soil results in negative pore water pressures which
draw in water to the piles to react. In soft, wet and relatively permeable
soils these suctions will be high only int he immediate vicinity of
the pile and for a short time. In drier clays of low permeability,
however, the suctions will be higher and will last far longer and,
since they increase the mean normal effective stress, the soil will
become stronger and morestable. This could be of considerable advantage
to failing slopes, if only to provide time for other stabilisation
mechanisms to come into operation. In addition these suctions will
result in:
2.5
Consolidation of the shear zone
Consolidation
of the clay int he remoulded shear zone of a failed slope can occuer
as a result of the negative pore water pressures (i.e. increased mean
normal effective stress) if they last long enough. This is discussed
in detail by Rogers and Glendinning (1993).
2.6
Pile strength
A final benefit
is the strength of the piles themselves, swich is said by several
authors to provide an incrase in bearing capacity. The same observation
could be applied to shear resistance along a shear plane in failing
slopes as long as pile durability and strain compability, both during
strength gain and after the pile has fully strengthened, are acconted
for.
3.
Stabilisation mechanisms
Two discints themes
emerge from the literature concerting the mechanism of stabilisation
and authors largely discuss one independently of the other. The first
of these is the idea of combined pile expansion and clay dehydration.
In general, the authors who propose this mechanism are using the lime
piles to improve the bearing capacity and settlement characteristics
of soft ground for foundations. The second theme concerns the migration
of calcium ions from the pile into the surrounding clay and its subsequent
stabilisation by lime-clay reaction. In general the authors who propose
this mechanism are relying upon the lime piles to stabilise failing
slopes in stiff clays or weathered shales and mudstones.
The literature will be discussed int he context of the stabilisation
mechanism listed above and, where possible, contradictory evidence
will be explained with the hindsight provided by the results of the
research at Loughborough University. Where one paper discusses more
than one mechanism, the work will be summarised under the first mechanism
and referred to thereafter.
3.1
Lateral consolidation
Several authors
describe the mechanism of lateral consolidation when using lime piles
for the improvement of soft soils, although the most notable exponents
are the Japanese. Kitsugi and Azakami (1982) describe the „Chemico-pile
method” in which granulated quicklime piles are constructed
by driving a closed-ended casing into the ground and then forcing
quicklime (using compressed air) into the cavity that is formed as
the casing is withdrawn. Improvements from field measurements are
given in Tables 1 and 2.
The main stabilising processes propounded in this paper are those
of dehydration and lateral consolidation caused by pile expansion,
which is said to „improve cohesion” int he soil. This
improvement is calculated by estimating the change in void ratio due
to the expansion, deter mined by multiplyng the reduced water content
by the specific gravity. This is used to find the increase in stress,
using the void ratio-effective normal stress relationship, which is
multiplied by a „strengthening factor” to reach the change
in „cohesion”. Reduction in vertical settlement is calculated
by considering that part of the settlement capacity of the soil is
taken up with lateral consolidation. Equations quantifyng these ideas
allow determination of the length of the piles required.
The source of the lateral movements seems to be entirely attributed
to the expansion of the lime. Piles of significantly increased volume
in comparison with the casings used have been observed int he field
and this is quoted as proof of the argument. While some expansion
of the pile diameter is inherent int he assumption of no discernible
net volume change, since the water from the soil becomes bound int
o the pile, the values of diameter quoted exceed this expansion. This
additional diametrical increase can, however, be attributed to the
driven casing causing lateral soil movements and the lime applied
under pressure, and subsequently compacted, expanding further the
cavity in ground as soft as the type mentioned. Thus physical displacement
caused by the construction process, rather than lime pile expansion
on lime hydration, occurs.
Wang (1989), from China, describes identical design equations to those
of Kitsugi and Azakami (1982), and yet neither paper references the
other. Tystovich et al. (1971) of Russia describe the use of lime
piles to aid compaction of 30 m of saturated loess. A closed-ended
tube system was used to create 250-500 mm diameter piles from compacted
lumps of quicklime at 2 m centres, causing the improvements shown
in Table 3. The pile diameter was observed to increase by 26-60%,
representing a volume increase of 160-260%, and radial cracking occured
int he surrounding loess. The volume increase is attributed to loess
compaction caused by the driving of the closed-ended tube and expansion.
Of the quicklime pile as a result of slaking.
Table 1.
Improvements in soft clay properties due to Chemico-Pile treatment
(after(Kitsugi and Azakami, 1982))
Dept
(m) |
Before
treatment |
After
treatment |
w2(%) |
y(Mg/m3) |
e |
Cu(kN/m2) |
w2(%) |
e |
Cu(kN/m2) |
5,5-6,1 |
122,2 |
1,34 |
3,34 |
12,0 |
163,0 |
3,39 |
78,7 |
7,0-7,3 |
88,3 |
1,47 |
2,39 |
15,0 |
86,4 |
2,31 |
52,1 |
10,0-10,8 |
98,3 |
1,42 |
2,75 |
19,0 |
79,1 |
2,09 |
56,2 |
14,0-14,3 |
85,6 |
1,49 |
2,28 |
24,5 |
76,1 |
2,06 |
59,8 |
* Notes: w
= water concent of soil; y = saturated unit weight; e
= void ratio of soil; Cu = undrained shear strength from
unconfined compression tests (Cu = 0,5 qu): qu
= undefined compressive strength.
Table 2.
Properties of the
Chemico-Pile (after(Kitsugi and Azakami, 1982))
Pile No. |
Diameter
(m) |
w2 (%) |
y (Mg/m3 |
qu (kN/m2 |
1 |
1,0-1,5 |
43,6 |
1,64 |
485 |
2 |
1,0-1,5 |
59,8 |
1,61 |
197 |
3 |
1,0-1,5 |
40,9 |
1,72 |
540 |
4 |
1,0-1,5 |
50,4 |
1,66 |
235 |
Mean |
1,0-1,5 |
48,7 |
1,64 |
378 |
* Notes: See notation
for Table 1.
Table 3.
Properties of loess treated by (Tystovich et al. (1971))
Pre-treatment
properties |
Water content |
24%-30% |
Plastic limit |
19%-21% |
liquid limit |
25%-30% |
Degree of
saturation |
95%-100% |
Effective
internal angle of friction Ø' |
17-20 |
Effective
cohesion c' |
4-5 kPa |
Properties
of loess 1 m from edge of piles |
Degree of
saturation |
61-72% |
Effective
internal angle of friction Ø' |
17-23 |
Effective
cohesion c' |
22-31 kPa |
Adding int he
volume increase caused by compaction of the quicklime ithe soft, weak
loess mass (i.e. forced lateral movement of the lime), the observed
diametrical increases appear to be adequately explained. The observed
cracks indicate a net volume loss, thus supporting the observations
made earlier.
Chui and Chin (1963) examined the expansive behaviour of quicklime
piles int he treatment of Taipei Silt for foundation purposes. The
experimental procedures adopted are unclear, although a photograph
indicates that the clay was compacted into boxes and small quicklime
piles were formed within it. An effective water supply and vertical
surcharge were thought nessecary for the full expansive force ont
he clay to be realised. This latter comment is important, the introduction
of water being recommended by others (although generally without justification
in this context).
Int he cases where water was added to a quicklime pile, for example
by surface inundation, then lateral consolidation caused by physical
expansion of the pile is likely. Another way in which this could,
in effect, occur is where water is removed via pores in a relatively
rigid soil structure (e.g. as would be caused in a weak sandstone),
thus creating the lateral stresses postulated. Otherwise lateral consolidation
by physical expansion caused by the lime should not be possible. In
soft soils the physical displacement of the soil by a driven tube
followed by compaction of the lime in the piles can cause physical
expansion and consequently lateral consolidation. Thus the effect
of soil densification can occur by several means, but the mechanisms
differ and the term lateral consolidation is, perhaps, confusing.
The successful use of lime piles in loess indicates that the densification
sought can be achieved without a significant clay content, this type
of improvement thus being applicable, for example, to alluvium in
general.
3.2
Water content reduction
The concept of
lateral expansion has often been couped with water content reduction
(?w). Kitsugi and Azakami (1982) developed the following equation
relating ?w to the original water content of the ground for estimation
of pile diameter and spacing.
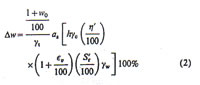
Where
?w = reduction in soil water content (%)
w? = original water content (%)
yt = wet density of soil in situ (t/m3)
as = area ratio of the Chemico-pile
as = ?d2/4s2
d = diameter of pile (m)
S = pitch of piles (square arrangement) (m)
h = equivalent of water absorption of lime column
yc = apparent saturated unit weight of lime pile (t/m3)
nt = porosity of slaked lime (%)
Ev = coefficient of volumetric expansion in slaking (%)
St = degree of saturation of slaked lime (%)
Yw = unit weight of water (t/m3)
The equation appears
to assume that water enters the pile solely to fill the void space
within it, and is in turn dependent ont he pile formation method.
Similar result may be achieved simply by calculating the amount of
water required to produce the same content int he pile as that int
he surrounding soil. Field result are compared with those calculated,
buta re only broadly comparable.
For ground with water contents of the orders quoted (50-150%) the
equation predicts large reductions. However, applied to overconsolidated
clays more typical of the UK the reductions are far more modest (?w
= 1% for w? = 30%, d=0,1 m and S=1,0m) necessitating large at relatively
small spacings for significant reductions. This is, in fact, what
the paper advocates, but the higher cost of lime production int he
UK could make it prohibitively expensive unless applied to wetter
alluvial soils for ground improvement since they can cause considerable
problems. In addition the facility for rapid ground improvement, which
this technique provides, wold influwncw the decision. Furthermore
for water content reduction there is no need for the soil to have
a significant clay content, as evidenced by Russian loess stabilisation
described later.
Kitsugi and Akazami (1982) present a case study in which lime piles
were used to reduce settlements beneath a 1.5-3.0 m high embankment.The
result show that the changes in water content are less significant
than those discussed earlier int he paper (Table 1) and that the water
content int he piles is signifacantly lower than that int he surrounding
soil. This could indicate an incomplete reaction. Factors affecting
water content transfer to the pile thus need further consideration.
The relationship between change in void ratio and water content does
not appear to hold in this case and therefore the dependent change
in undrained shear strength (?cu) cannot have been accurately calculated.
Since ?cu was shown to increase with depth, this could indicate that
the effects recorded were due to vertical consolidation caused by
reduced pore water pressures increasing the mean normal effective
stress. However insufficient data are presented for full validation
of the equations or explanation of observed phenomena.
Tystovich et al. (1971) of Russia describe how water content reduction
is effected by the heat of the slaking reaction causing evaporation.
They state that the best results are archieved in porous soils such
as saturated loess. These conditions would undoubtedly enhance water
movements within the soil, thus promoting a more complete and rapid
reaction. Int he field trials steam was observed and cracks up to
40 mm wide and 480 mm long developed around the 250-500 mm diameter
piles. Temperatures observed at the centre and edge of the piles peaked
at 390o and 50 Co , respectively around 4 h after construction. These
were remarkably similar to measurements arround 1000-1500 mm diameter
quicklime piles in peat having water content of 400% to 460% (Kitsugi
and Azakami, 1982). Samples teken 1 m away from the pile edge had
a significantly reduced degree of saturation and increased strength
parameters (Table 3). A plate load test carried out int he area containing
the pile group deformed substantially less than that on untreated
ground. It is unclear at what depth the tested samples were taken
and, hence, if the water content reduction occured over the entire
pile length or if it was a surface effect attributable to the steam.
The volume of water used up int he slaking reaction is thus simple
to calculate, the calculation showing that large diameter piles at
relatively smallspacings, as used in the soft soil treatment of the
far east, are necessary to generate large water content reductios.
Large piles and a source of rapidly availabla water (a sin soils with
a high permeability and/or high water content) will create the conditions
for significant steam generation and increased water loss from the
soil. The presence of clay is not necessary, and since is would reduce
the permeability of the soil it could prove a hindrance to stabilisation
by this mechanism.
3.3
Clay-lime reaction
Many authors propose
that the pozzolanic clay-lime reaction, initiated by the „migration
of lime” from the piles into the surrounding soil, is a major
stabilising process int he treatment of failing slopes, and this is
discussed below. Kitsugi and Azakami (1982) discuss how the „lime”
content int he ground diminishes with distance from the pile as a
result of thier studies of soft soil improvement. However, it is likely
that lime being forced into very soft ground under pressure would
tend to cause significant displacement of the surrounding soil and
yet no account is apparently taken of this Tystovich et al. (1971)
also include chemical reaction between the relatively small quantity
of clay held within (soft, wet) loess and migrating lime as a stbilishing
mechaninsm, but present no supporting data. Chui and Chin (1963) similarly
mention the possibility of clay-lime reactions int he context of soft
soil improvement, altough again without alaboration. The literature
on soft soils thus provedes few leads.
Several American authors refer to the mehanism in slope stebilisation.
Handy and Williams (1967) describe a case study in which 150 mm diameter
quicklime piles were successfully used to stabilise a failing clay
fill slope where more conventional treatments had failed (Fig. 4).
The clay fill had a mineralogy of predominantly calcium montmorillonite,
properties int he active slippage zone ofØ = 17o, c’=4
kPa nd a water content of 27,2%. Design methods were entired based
ont he prdicted lime migration distance and ont he soil typein relation
to the subsequent clay-lime reaction. The piles were constructed on
a 1,5 m grid to penetrate the zone situated within a perched water
table. Holes ware augered, and quicklime was poured in and „watered”.
Sampling indicated a water content reduction of 4% after 3 months,
although the sample locations were not described. After 1 y the unconfined
compressive strength had risen relative to the 3-month strength by
approximatelly 55%. Acid soluble aluminate and silicate were said
to be indicative of a reaction having occured 300 mm from a pile.
After 2 y a soil sample taken 300 mm away from a pile and 2 m below
its top had Ø’ = 21 o and c’= 9,5 kPa. No lime
movement could be detected above the water table, thus indicating
the need for water int he movement of the lime. Tests ont he lime
piles indicated that very little of the lime had reacted. A subsequent
slip occured underneath the treatment area, highlighting the need
for comprehensive analysis of the unstable region prior to treatment.
Over the 2-y period after treatment, cracks int he houses founded
ont he slipped area closed up and no further cracking took place.
This could be explained initially by the expansion of the piles due
to the introduction of additional (i.e. external) water. Int he longer
term, the strengthening of the lime placed througn the shear zone
prevented further movement. If some hydration of the piles was caused
by water from the softened zone around the slip plane, improvement
of properties could have been affected by dehydration and consolidation
(see Rogers and Glendinning, 1993). The improved soil properties could
be explained by lateral consolidation, although the time dependence
of the improvement is difficult to attribute, or even natural soil
property variation. However lime migration assisted by hydraulis transport
(„watering” remains likely.
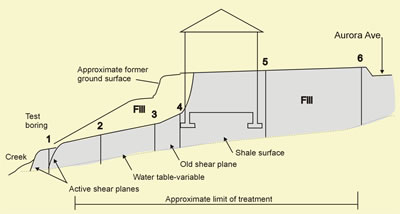
Fig. 4. The use of lime piles in slope stabilisation in Iowa ( after
Handy and Williams, 1967).
The most interesting
result is the measurement of acid soluble aluminates and silicates,
which are indicavite of the clay-lime reaction. The equation of Davidson
et al.(1965):
L = kt0,5 (3)
Used to calculate
rates of movement appears to be invalid when there is a movement of
water (see later discussion). However, purely considering permeabilití
and water flow, a rate of ion migration of the order quoted would
indicate a coefficient of permeability for the clay of 5x10-9 m/s,
which seems reasonable. The anomaly appears to be that the treatment
was designed ont he basis of the quantity of lime required to promote
a chemical reaction and very little of the lime had reacted. As it
is believed that a certain pH (>12.4), hence concentration of „lime”,
is required before aluminates and silicates go into solution, it is
questionable as to whether a reaction was occuring. It may be possible
that the acid used int he test is breaking down the clay and giving
these results.
Anon (1963) and Lutenegger and Dickson (1984) describe similar usage
of lime piles where a flow of water was thought to be necessary to
promote lime migration.
As part of expensive Indian studies, Chummar (1987) reports model
laboratory experiments on marine and moderately expansive clays using
20 mm diameter quicklime-sand piles at 200 mm spacing. He also describes
their successful fielduse, including the stabilisation of a slip plane
in a lateritic clay embankment. Unfortunately no design criteria are
discussed. Further field trials are described by Shanker et al. (1989).
Again, the flow of water to transport the lime was felt essential,
so the piles were „watered” for 3 weeks after installation.
Also, a 50 mm diameter sand core was provided int he soil-lime pile
as it was thought this would aid drainage.
Ayyar et al. (1989) examined the effect of pile shape on its efficiency
via a combination of field trials and laboratory trials on clay compacted
into a 560 mm diameter, 850 mm deep tank. The mineralogy of the clay
used was predominantly smectite, with liquid and plastic limits (LL
and PL) of 60 % and 21 %, respectively. Augered holes were filled
with quicklime and allowed to reach equilibrium with a thin layer
of water ont he surface. Pile sizes were calculated so as to achieve
2 % by dry weight of lime. Clay samples taken after 1, 2, 3 and 6
weeks were analysed for calcium content, shearstrength and water content.
The study concluded that more calcium than was required for ion exchange
had migrated into the surrounding soil, although again without justification
or even information on how far the ions had migrated.
Venkatanarayama et al. (1989) used similar laboratory models with
surface inundation to study the effects of pile spacing. Tests on
samples taken from between the piles demonstrated that smallaer spacings
gave greater degress of improvement although once more detailed data
were lacking. One interesting aspect is their attempted comparison
between the effects of the piles and traditional mixing. It is, however,
considered inappropariate by the current authors to compare directly
percentages of lime between the different treatment methods and this
graph should be used with caution. Using a mix technique, the modification
process relies partly on pulverisation, flocculation and recompation
to achive improvements in plasticity, strength and workability. The
cementation reactions then bind small „lumps” of (modified)
clay together. These processes do not happen via ion migration. Improvements
from the clay-lime reaction must be derived solely on a molucelarlevel,
the main stabilising mechanism being derived from other processes
entirely.
Brandl (1981) describes lime pile usage in Austria since 1968. Laboratory
tests and field trials have shown that long-term strength is derived
from diffusion of lime from the piles. Their efficiency for any particular
application is thought to be controlled by the clay mineral content,
ion exchangeability and the presence of movable silica. The most suitable
soils are thought to be „loosely packed” and of low plasticy,
since they have an increased natural permeability and remove the risk
of rapid cementing reactions preventing further diffusion by „sealing
in” the lime. Piles of 80-500 mm in diameter at spacings of
0,5-3,0 m (depending on soil charasterics) have been employed to give
changes in Ø’ of as much as 15o. No long-term data were
available, but Brandl doubts if the reactions can be reversed with
time.
Ruenkrairergsa and Pimsarn (1982) describe the stabilisation of a
loose clay shale fill embankment in Thailand. Hand augered, 150-mm
diameter holes were formed on a 3-m grid through to the natural ground
(Fig. 5). Lime and water were simultaneously poured into the holes,
the mixture being topped up four times daily for 2 months. Pile construction
occured immediately prior to the rainy season as it was thought that
limemigration would not occur without the flow of water. Lateral movement
was monitored by means of aluminium rods driven through the madeground
into the natural ground below (Table 4). Shear strength was monitored
in situ using an „Iowa borehole shear device” (Hnady and
Fox, 1967), water content and consistency limits being measured ont
he samples thus recovered (Table 5). Samples were taken immediately
after the rainy season.
The authors state that lateral movement has been reduced, although
the result show that movement is still occuring. The strength changes
are attributed to improved drainage caused by the lime migration.
The significance of the results is difficult to ascertian since no
„pre-treatment” lateral displacement or climatic data
are available: a drier rainy season eould reduce movement if water
were the trigger of the slip. The plasticy index reduced from a range
of 20-30% to an average of 12% due to a rise in PL, a change that
could reasonably be attributed to a clay-lime reaction (Rogers and
Glendinning, 1996a). Although apparently successful, the topping up
of lime slurry in each hole on 240 occasions results in a extremely
labour intensive operation and one that is likely to prove fruitless
in intact clays.
Fosh and Kinter (1972) carried out a laboratory study using high calcium
hydrated lime to treat small blocks of montmorillonitic and kaolinitic
clay.Free calcium ion concentrations were detected by means of acid
extraction and titretion with Ethylenediaminetetracetic Acid (EDTA).
Migration was found to be limited to less than 40 mm after 180 days.
They concluded that water movement and ion migration were separate
processes, with the most concentrated solution of limecontributing
most lime to the soil independent of clay water content.
Katti and Gupta (1970) studied lime migration in Black Cotton Soil
(LL = 80%, PL = 40%) using models of quicklime piles in soil and soil
piles in quicklime. Unconfined compressive strength and shrinkage
limit were used as measures of migration.
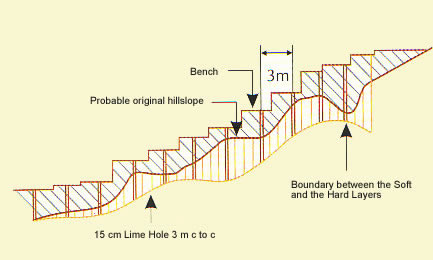
Cross
section of clay shale embankment at km. 115-500 on the Chiangmai –
Chiangdao Fang National Highway.
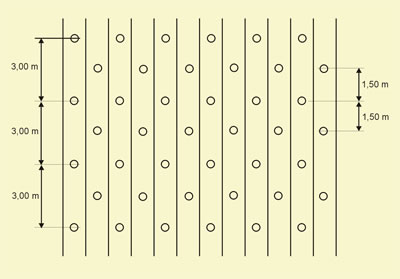
Fig.
5. Application of lime piles to a clay shale embankment (after Ruenkrairergsa
and Pimsarn, 1982).
It was concluded
that lime had travelled to the centre of a 150 mm soil specimen after
120 days. Although both in extreme proved detrimental, migration was
thought to be aided by lower water contents and higher soil densities
via promotion of capillary action.
Noble and Anday (1967) constructed 25-mm diameter lime paste piles
within 150-mm diameter compacted clay speciments of unknown mineralogy.
The tops were plugged with clay and the samples sealed. Migration
was monitored by leaching soil samples with acid titreting with EDTA
to determine the free calcium ion content.
Table
4.
Date |
Elapsed
time (days) |
Distance
from observation stakes to reference stakes (m) |
Distance
increased from original value (m) |
Line
1 |
Line
2 |
Line
3 |
Line
4 |
Line
1 |
Line
2 |
Line
3 |
Line
4 |
20 may 1977 |
0 |
15.005 |
15.560 |
15.620 |
15.184 |
0 |
0 |
0 |
0 |
1 april 1978 |
311 |
15.024 |
15.571 |
15.633 |
15.184 |
0.019 |
0.011 |
0.013 |
0 |
1 december
1978 |
551 |
15.035 |
15.600 |
15.690 |
15.200 |
0.030 |
0.040 |
0.070 |
0.016 |
17 June 1979 |
748 |
15.040 |
- |
15.724 |
15.204 |
0.035 |
- |
0.104 |
0.020 |
Lateral movement of a clay shale slope after lime pile (after (Ruenkrairergsa
and Pimsarn, 1982))
Table
5.
Test
depth (m) |
Water
content, n (%) |
|
Cohesion,
c (kN/m2) |
|
Angle
of shearing resistance Ø (deg) |
|
1977 |
1979 |
1977 |
1979 |
1977 |
1979 |
Range |
Avg. |
Range |
Avg. |
Range |
Avg. |
Range |
Avg. |
Range |
Avg. |
Range |
Avg. |
1 |
30.2-55.0 |
43.4 |
27.4-48.1 |
37.4 |
-6.0 |
0-19.3 |
7.1 |
0-17.9 |
7.2 |
+0.1 |
22.0-44.5 |
33.9 |
29.5-48.0 |
39.8 |
+5.9 |
2 |
30.5-51.2 |
42.6 |
23.5-53.8 |
38.3 |
-4.3 |
0-37.9 |
12.9 |
0-30.3 |
13.9 |
+1.0 |
22.1-39.4 |
31.2 |
28.0-47.0 |
39.3 |
+8.1 |
3 |
31.1-52.8 |
42.4 |
26.8-54.0 |
38.9 |
-3.5 |
0-35.0 |
11.3 |
4.1-27.6 |
15.5 |
+4.2 |
20.0-40.2 |
32.3 |
31.5-46.5 |
38.8 |
+6.5 |
4 |
28.5-54.5 |
42.5 |
22.3-51.8 |
38.0 |
-4.5 |
0-38.6 |
12.2 |
3.4-29.7 |
1.57 |
+3.5 |
20.1-44.0 |
33.3 |
27.5-47.6 |
37.5 |
+4.2 |
Changes
in water content, cohesion and angle of shearing resistence after
lime treatment (after [Ruenkrairergsa and Pimsarn, 1982])
(+) means increase,
(-) means decrease; 100 kN/m2 = 14.5 lb/m2.
Ions migrated
45 mm, the limit of the experiment, in 44 days. At shorter distances
ion concentrations increased over 99 days. Some of the early readings
indicated that migration had occured, whilst later data at the same
distance did not. This was attributed to migration occuring in paths
of lower density material. Although not mentioned, it is possible
that free calcium ions became unavailable due to cation exchange.
It is thus widely reported reported that lime migrates from the piles
and reacts with, and hence stabilises, the surrounding ground. In
order for this to occur both calcium ions and hydroxyl ions must migrate
through the clay. Saturation of the system by calcium ions is neseccary
for maximum cation exchange ont he available exchange sites of the
clay minerals. Hydroxyl ions produce the highly alkalinic (pH >
12,4) conditions required for dissolution of silica and alumina prior
to the formation of calcium silicate hydrate and calcium aluminate
hydrate, the gels that crystallizse to cause stabilisation. Laboratory
experiments have shown that the migration in clays is restictec to
a relatively small distance, as would be expected from consideration
of the operation of clay barriers. Water transport of ions from quicklime
piles inintact clay dous not appear to be a valid mechanism, and indeed
it is suggested that capillarity provedes the mechanism such that
drier clays are better in this respect.
The actual mechanism is probably a combination of ion diffusion and
mass transport depending on water flow conditions. With respect to
diffusion, a possible explanation for the short distances observed
is that it is controlled by ion exchange. Ions may radiate away from
the pile by a process of ion exchange, an equlibrium position being
achieved when the potential for ion exchange is balanced by a reverse
osmotic potential. The potential for ion exchange must, in turn, depend
on mineralogy. In cases where lime slurryhas been used to create the
piles, migration over significant distances is reported, presumably
as a result of hydraulic transport. „Watering” of quicklime
pileshas also been suggested in order to improve hydraulic transport
of ions. The measurements of ion migration are, however, suspect in
many cases and in some cases migration is claimed without measurements
to support the claim. The self-limiting nature of the reactions, in
which stabilisation of the soil adjacent to a pile inhibits further
migration, must also be considered int he migration process. These
ideas are expanded further by Rogers and Glendinning (1996b).
3.4
Reduction in pore water pressure
Although not mentioned
int he literature, this mechanism has been observed during the research
at Loughborough University. Generation of negative pore water pressure
is caused by the strongly hydrophilic quicklime int he piles drawing
in water from the surrounding soil. Reductions of the order of 20
kPa have been observed in both laboratory trials and, importantly,
field measurements. This mechanism represents an important short-term
process.
3.5
Consolidation of the shear zone
Again not mentioned
int he literature, overconsolidation of remoulded clay along a shear
plane, or zone, as a consequence of the negative pore water pressure
leads to increased strength. The caly int he shear zone is particularly
susceptible to this form of improvement since water contents are typically
higher than int he remainder of the soil. Improvements are small,
but when applied to shallow slips they are significant in terms of
stability. This is discussed in greater detail in a later section.
3.6
Pile strength
Kitsugi and Azakami
(1982) are the only authors who specifically mention the strength
of the piles themselves, attributing improvements in bearing capacity
of soft soils mainly to pile strength.
An average strength of 431 kN/m2 is quoted. Additivies of calcium
silicate and/or calcium alluminate are pre-mixed with the quicklime,
presumably to improve strengtn. Pile strength is said to depend upon
the confining pressure provided by the surrounding soil. Such lateral
support will increaseconsiderably with depth and thus the degree of
vertical confinement of the clay close to the surface will be important
in how such piles should be designed. In practice it will be the pile-soil
systemthat resist the applied stress and an equilibrium condition
will be reached.
4.
Concluding discussion int he context of UK applications
The literature
has thus provided much evidence of the cussess of lime piles in treating
both soft groung to improve its bearing capacity and slopes to improve
their stability. The mechanisms of stabilisation postulated by authors
from several different countries are in some cases incorrect, and
a significant proportion of the literature ont he subject is contradictory
or misleading. Thus although the technique is of value, the need for
a comprehensive programmme of researc, both int he laboratory to isolate
the stabilisation mechanisms operating and int he field to prove the
techniques, has been established.
The researc at Loughborough University examined the application of
quicklime piles to stabilise slope failures and this will be discussed
briefly here int he context of the above stabilishing mechanisms.
The conclusions are draw from laboratory data and, more especially,
observations of field performance over a period of more than 4 y.
The research concentrated upon the shallow failures described by Perry
(1989), 95% of which were less than 2 m deep and thus had effective
normal stresses acting ont he failure plane of typically 10-40 kPa.
No such considered research programme has been published ont he application
of quicklime piles to soft soil improvement, and indeed this paper
represents the first comprehensive critique of this promising potential
application.
When analysing
shallow slope failures it was evident that small changes in the effective
cohesion (c`) dramatically altered the factor of safety of the slope
. Although it can be argued that c` effects in soils are artificial
and c` ?provides a por basis for analysis reliance on c` becomes more
acceptable if the increase in strength is considered as a ”
cementation” rather than a ”cohesion”. In the case
of quicklime piles this additional c` is relatively large and derives
from different sources , the effects of which are superimposed to
generate significant improvements in safety factor at different times
after construction . It is with this in mind that a definitive list
of stabilising mechanisms was developed .
In the overconsolidated clay cuttings and embankments that are typical
of the UK ,significant expansion of quicklime piles is not thought
to occur due to the degree of confinement and relatively low initial
water content . Even if some degree of external water ingress occurs
, for example via the system of radial cracks generated by rapid clay
dehydration . The high degree ofconfinement will tend to cause densification
of the lime within the pile .Reducton in water content of the clay
mass as a whole is also likely to be relatively modest . Field data
show it to be confined to a small annular zone around each pile ,with
differential dehydration causig radial cracking . Migration of lime(i.e.
Ca2 + and OH – ions)in any significant quantity is likely to
20-50 mm from the pile or from lime-filled cracks. (Greater migration
of Ca2+ ions in the much longer term should not be discounted , even
though direct evidence is currently lacking , since Quigley et al
., 1990, have indicated this to occur in work associated with landfillv
liners.) This necessarily limits the facility for subsequent clay-lime
reaction. The reduction in pore water pressure generated by quicklime
piles is relatively high.These suctions immediatety increase the stability
of the slope, in the same way that considerable stability exists due
to the high stress relief suctions that are generated whet creating
slopes. Overconsolidation of the shear zone is dependent upon site
–specific ground conditions, but improvements in effective cohesion
would be expected to be of the order of 1-5 kPa if complete and effective
overconsolidation were to occur. Significant pile strength is developed
with time and is dependent (in part) upon the degree of confinement
and (strongly) upon iniital clay water content. Undrained shear strengths
of 400-500 kPa have benn recorded in the field after 2 y and in the
laboratory (i.e. under ideal conditions) after 4 months . In soft,
very wet alluvial soil found in an isolated buried stream valley,
however, no apparent strength gain occurred in piles constructed without
pressurised insertion of the quicklime.
For the above mechanisms to be relied upon, the technique should be
restricted to seft-supporting clay soils in which relatively dri augered
holes can be constructed, unless alternative construction techniques
are developed. Hawever, there in no reason to believe that the application
of lime piles to soft soils could not be adopted in the UK, albeit
that researrch is not currently available to prove its efficacy.
Acknowledgment
The research programme
conducted at Loughboroughm University was funded jointly by four industrial
partners (Geotechnics Ltd, Cementation Piling and Foundations Ltd.,
British Waterways and Buxton Lime Industriest) and the Science and
Engineering Research Council(now the EPSRC) via the LINK Scheme in
Transport, Infrastructure and Operations. This funding, and the considerable
assistance of the staff of the above organisations, is gratefully
acknowledgedged.